New enzyme optimises material cycles
A research team led by Marburg microbiologist Tobias Erb has found a new way to effectively fix CO2 and transfer it into the cell metabolism. A completely new enzyme was designed for this purpose and optimised through evolution in the laboratory.
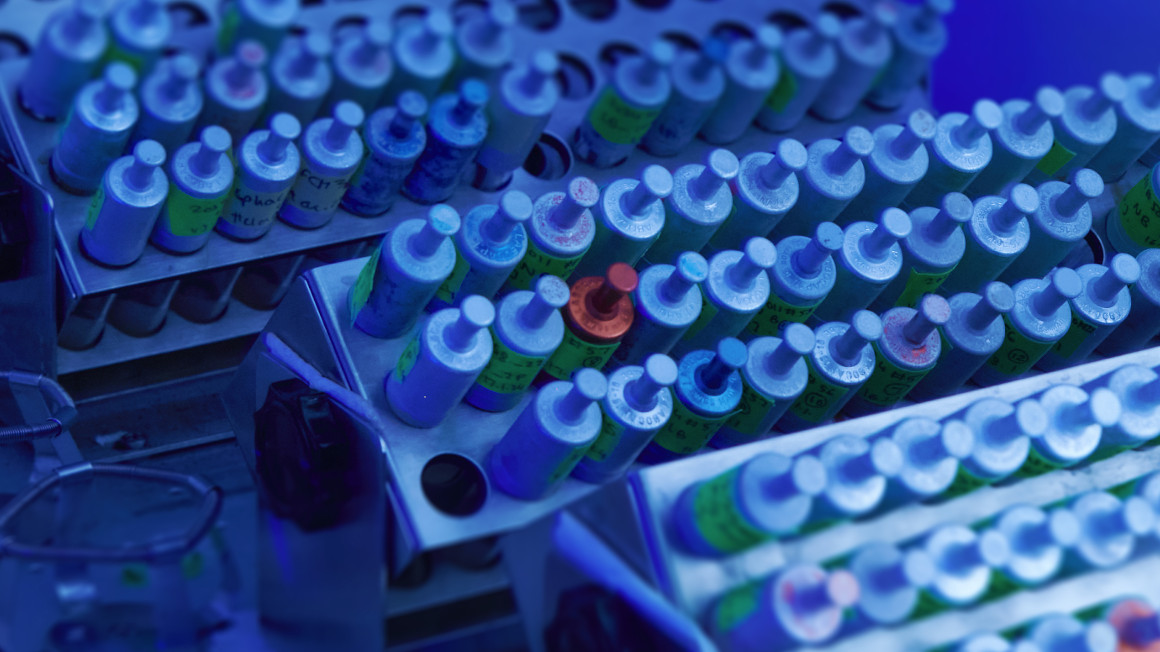
As an intermediate product of many metabolic pathways, acetyl-CoA is a central component in the production of vital biomolecules. The molecule binds carbon dioxide (CO2) and is therefore crucial for how efficiently the greenhouse gas is incorporated into metabolic processes in order to ultimately produce biomass.
An important step in the conversion into other metabolic products is the production of so-called C3 bodies - intermediate products such as pyruvate with three carbon atoms. Naturally, these metabolic pathways take place in numerous steps and require a lot of energy. With the help of synthetic biology, Tobias Erb's team at the Max Planck Institute for Terrestrial Microbiology is trying to make this process of CO2 fixation as efficient as possible.
Synthetic biology improves CO2 utilisation
The researchers recently achieved this with the development of a synthetic cycle for CO₂ fixation in bacteria. In the scientific journal Nature Communications, they now report another significant advance - the development of a new enzyme that creates a more direct link between acetyl-CoA and pyruvate.
According to the authors, this study is a successful example of how the mechanisms of metabolism and evolution in living cells can be used to optimise a desired property for applications in synthetic biology and biochemistry. Improved enzyme variants can only be found by combining the two.
From blueprint to functioning enzyme
In synthetic biology, metabolic pathways are developed and then tested in the laboratory. This is exactly what the Marburg team did: they designed a metabolic pathway to bind additional CO₂ as directly as possible and without detours. To do this, they designed a so-called lactyl-CoA mutase, which has not yet been described in nature. In their search for suitable candidates, they found what they were looking for in an enzyme database: The enzyme was indeed able to convert the desired metabolic steps – albeit very slowly. So the enzyme first had to go to a training camp, as the researchers aptly describe. In a kind of evolution in the laboratory, they were able to gradually optimise the enzyme and introduce it into a bacterial strain.
‘To ensure that the newly acquired ability was not immediately lost again due to further mutations, we first coupled the growth of a modified Escherichia coli bacterium to the desired enzyme activity,’ says first author Helena Schulz-Mirbach, describing the challenge. ‘The fact that we were able to develop a strain that utilises this slow enzyme for its growth was not a given.’
Targeted evolution for enzyme optimisation
In order to further improve the enzyme, mutations were specifically created in the bacteria and then the desired properties of the strain were selected. This so-called adaptive laboratory evolution ultimately resulted in variants of the lactyl-CoA mutase that worked faster and at the same time led to better growth of the strain. This made it possible to allow the enzyme to work in vitro, i.e. in a simplified chemical process outside the bacterium. The improved enzyme thus performed five to ten times better than the natural precursor.
Wide range of possible applications
Next, the Marburg researchers want to further develop the designed and optimised lactyl-CoA mutase in order to make the enzyme even faster and therefore more versatile. The newly created metabolic pathway between acetyl-CoA and pyruvate has a wide range of potential applications. For example, the production of 3-hydroxypropionate can be facilitated, which in turn serves as a precursor for the sustainable bioplastic polyhydroxybutyrate (PHB). But that's not all: ‘We also want to learn more about the enzyme itself,’ says Schulz-Mirbach. ‘We know which mutations have improved its activity, but we don't yet know how they do this. If we can elucidate the structure of the enzyme, we can find out more about the reaction mechanism and understand how the mutations improve the enzyme.’
chk