1) Alternatives to the cell as a biofactory
In industrial biotechnology production, microorganisms, but also animal and plant cell cultures, are usually used as living factories to produce proteins and other metabolic products such as antibiotics, amino acids or biopolymers.
Another process has established itself as an alternative approach to these cell-based processes: cell-free biosynthesis. Cell-free production systems dispense with the use of living cells. The synthesis of biomolecules such as nucleic acids or proteins takes place artificially using all the necessary components in a reaction vessel - i.e. in vitro.
Cell-free production systems have numerous advantages over cell-based processes. The most important is the considerably lower complexity of the systems: microorganisms or cells are cultivated in bioreactors at considerable material and energy expense, since in addition to the actually desired biomolecule they also permanently produce a large number of vital, cellular proteins and these can lead to numerous by-products with their activity. If one detaches themselves from the complex system of the cell and concentrates only on the relevant synthesis steps, special proteins or other biomolecules can be produced easier, significantly more efficient and in higher purity.
Experts distinguish between two approaches:
• Cell extract-based systems: All components of the cellular machinery necessary for biosynthesis are combined in one reaction vessel. For this purpose, so-called lysates are obtained by cell disruption. They are used in particular for protein synthesis in the laboratory (chapter 2).
• Enzyme-based systems: Instead of whole cells, only the necessary isolated enzymes are used for substance transformations - these then catalyse a specific reaction (chapters 3 and 4).
Since, unlike cell-based systems, the steps of cell multiplication and disruption are omitted, cell-free production is in many cases cheaper, more flexible and considerably faster. This dossier explains the potential of cell-free bioproduction in research and development, describes limitations of this approach and presents important innovative concepts and applications.
We would like to thank the cell-free biotechnology experts Dr. Stefan Kubick (Fraunhofer IZI-BB) and Prof. Dr. Volker Sieber (TU Munich) for the scientific fact check of this dossier.
Page 2 of 5
2) Cell-free biosynthesis of proteins
Cell-free protein synthesis systems are based on the use of the cell's internal molecular machinery to produce proteins. This includes ribosomes, translation factors and enzymes. They accomplish the processes of transcription and translation necessary for protein production.
Two steps to becoming a protein
Protein biosynthesis is a two-step process in which the genetic information stored in the DNA is converted into a protein. During transcription, the enzyme RNA polymerase produces a single-stranded complementary transcript of the DNA sequence of the gene from nucleotides, the so-called messenger RNA (mRNA). Translation then takes place at the ribosomes, in which the nucleotide sequence is translated into an amino acid sequence. Defined by the triplet code of the nucleotides, amino acids are bound to an amino acid-specific transfer RNA (tRNA). In the ribosomes, the amino acids are then linked to form a chain by peptide binding. As soon as this polypeptide chain leaves the ribosome, it begins to fold in a specific way due to chemical and physical interactions.
How do you access the cellular protein synthesis machinery in order to use it biotechnically? With the help of special digestion methods, the membranes surrounding the cells are dissolved and the cell contents, the so-called extract or lysate, are used to produce the desired protein. In many cases, the lysates come from model organisms such as the bacterium E. coli; plant cells (wheat germ extract, tobacco) and animal cell cultures (hamster cells, insect cells) are established for the production of more complex proteins. To produce the relevant components of a cell-free system, therefore, a cell-based step must always be carried out upstream, i.e. in which cells are cultivated and their contents extracted.
In order to produce a protein in vitro, the lysate is placed in a reaction vessel together with the protein-coding DNA sequence, energy-providing substances and amino acids. Now the synthesis proceeds on its own. Within a few hours, the "finished" protein is obtained. The purification and recovery of the protein produced from the reaction mixture are comparatively simple. Overall, this is more resource-efficient, faster and cheaper than cell-based methods. In addition, in vitro syntheses can be carried out in a highly parallelised and automated manner.
Cell-free beginnings
The earliest basis for cell-free production systems dates back to the 1960s, when Marshall W. Nirenberg and Heinrich Matthaei were able to show with the so-called Poly-U experiment that certain RNA molecules function as matrices that cells can use to produce proteins. The first syntheses of larger protein quantities in cell-free systems took place in the late 1980s in the so-called CECF process (Continuous-Exchange Cell-Free). Since then, research has intensively investigated which parameters limit cell-free biosynthesis and how it can be optimised. Commercial systems are available in prokaryotic organisms (bacteria and archaea) as well as for systems from eukaryotes (human, animal, plant and fungal cells). Modern cell-free production platforms deliver up to several milligrams of protein per millilitre.
Application areas in research and production
Cell-free protein synthesis has established itself as an important tool in basic biomedical research. Cell-free systems are mainly used for proteins that cannot be produced with conventional cell-based systems. These are, for example, membrane proteins that can be provided in high purity for pharmaceutical research to elucidate their structure or functional mechanism in milligram quantities.
In addition, many pharmacologically interesting proteins that are urgently needed for research have a toxic effect on cells in higher concentrations. As a result, important protein classes cannot be produced at all or only in very small quantities. Cell-free biosyntheses can therefore show their advantages in the production of cytotoxic and poorly soluble proteins.
Since in vitro systems can be handled very flexibly and events in the reaction vessel can be accessed directly, they are also used in protein engineering. This term describes a mix of methods that leads to the exchange of amino acids of the protein and thus optimises its enzymatic properties for use in certain processes.
Synthetic biology researchers use amino acids that are modified or non-proteinogenic, i.e. not used for protein synthesis in natural systems. However, they can bring desirable chemical properties, which is why cell-free biosyntheses make good use of them.
Another advantage for research is the possibility of introducing labelled tRNAs into protein synthesis. In this way, the researchers can later visualise in their studies where the reporter proteins labelled in this way move in the cells and with which other proteins they interact.
Compared to production systems from bacteria or archaea, those from animal and plant cells offer some advantages. For example, so-called post-translational modifications of the proteins can be carried out. Chemical groups of the proteins can be changed or added in order to specifically adapt the effect or binding specificity of the proteins. Such cells are therefore suitable for the production of biopharmaceuticals, especially antibodies. Cell-free systems are also suitable for the production of antibody drug conjugates - combination molecules that are used as cancer therapeutics, for example. Antimicrobial peptides and cytokines can also be produced cell-free.
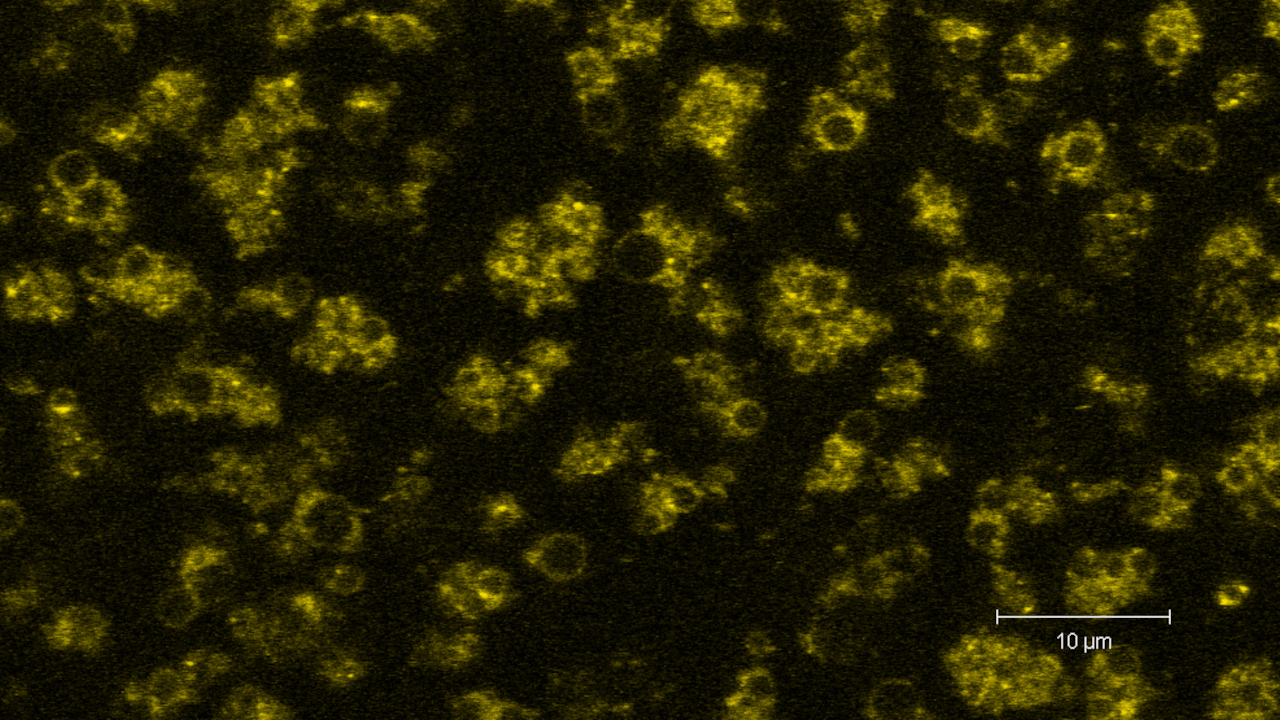
Challenges
One challenge is to ensure that the peptide chains fold in the same way during protein synthesis as they would in the cell - otherwise the protein cannot fulfil its normal function. In addition, continuous processes must be developed that guarantee a balanced supply of expensive energy-providing substances (ATP), cofactors (recycling) and individual building blocks (amino acids). This is particularly relevant for the scale-up of cell-free bioproduction processes to industrial scale. Cost competitiveness with cell-based production of biopharmaceutical proteins has only been achieved in individual cases.
Activities in science and business
An important contribution to bringing cell-free biosyntheses into practice was made from 2011 to 2014 by the Fraunhofer lead project "Cell-free bioproduction", in which eight institutes participated. The result is a technology platform with which the researchers have produced antibodies, among other things. The project participants were able to reduce the costs by a factor of ten compared to conventional methods - on a small and medium production scale. The Federal Ministry of Education and Research (BMBF) funded the initiative with around 14.8 million euros, with further funding provided by the Fraunhofer-Gesellschaft.
Examples of companies that focus on cell-free bioprotein synthesis are Kykeon Biotech and LenioBio. The former is a spin-off of TH Wildau and produces recombinant proteins, including antibodies. Leniobio uses a Fraunhofer IME technology for eukaryotic cell-free protein expression and is dedicated to the synthesis of difficult-to-make proteins for all sectors, including biomedicine, industry and agriculture.
Page 3 of 5
3) Cell-free enzymatic single-step reactions
Cell-free biocatalysis is similar in many respects to cell-free bioprotein synthesis. The difference is that proteins are not produced here, but the catalytic function of isolated enzymes is used for substance transformations. The components required for the desired substance conversion are introduced into the cell-free reaction system in which the desired substance conversion then takes place.
Purely enzymatic catalyses have already been established in industrial production for many years, especially when it comes to substance transformations involving an enzyme or a few enzymes. Many industrially relevant enzyme processes take place under conditions that are not suitable for microorganisms (such as starch saccharification, which takes place at 80 to 105 degrees Celsius).
As with cell-free protein synthesis, the enzymes used must first be produced - usually in cells. This must be taken into account in economic considerations and life cycle analyses. In cell-free enzymatic catalysis, experts distinguish between two processes: the single-step reaction, in which a specific enzyme catalyses one reaction step, and the multi-step reaction, in which an enzyme cascade catalyses a complex, multi-step synthesis process (more on this in chapter 4).
Enzymatic single-step reactions
The food industry in particular has been using individual enzymes cell-free for decades. Most of these are hydrolysing enzymes, for example proteases, amylases, β-glucanases or pectinases. They help in starch saccharification, clarify fruit juices, produce prebiotics or prevent baked goods from becoming stale quickly. These enzymes are already offered in very large quantities at relatively low prices (10 to 50 euros per kilogram) by different manufacturers.
While the examples mentioned so far have liquid reaction environments, there are also cases in which enzymes act in immobilised form, i.e. the enzymes are fixed on carrier materials or embedded in gels, for example. For example, xylases separate lignin in paper production, which makes it easier to bleach the paper and improve the fibre structure.
Especially since the demand for biofuels is growing, cell-free enzyme reactions play a role here as well. Cellulases make it possible to utilise the important biogenic raw and residual material lignocellulose. All these examples have in common that the enzymes involved are comparatively robust and usually do not require cofactors or other high-value substances for the respective catalyses. Cofactors are non-protein components that are indispensable for the activity of an enzyme.
Cell-free vaccine production
COVID-19 mRNA vaccines have had and continue to have a significant role in the management of the corona pandemic. The production of mRNA molecules on an industrial scale also relies on a cell-free bioproduction step. The enzyme RNA polymerase is of central importance here. First, the blueprint for the spike protein of the SARS-CoV-2 virus is incorporated into a plasmid and amplified millions of times in bacteria. After a purification step, the DNA is divided into individual strands. Now the RNA polymerase comes into play. With the help of the required molecular building blocks, it transcribes the DNA into a complementary mRNA strand. According to the manufacturer BioNTech, this process takes three to four days. The product is purified and then packaged in lipid nanoparticles.
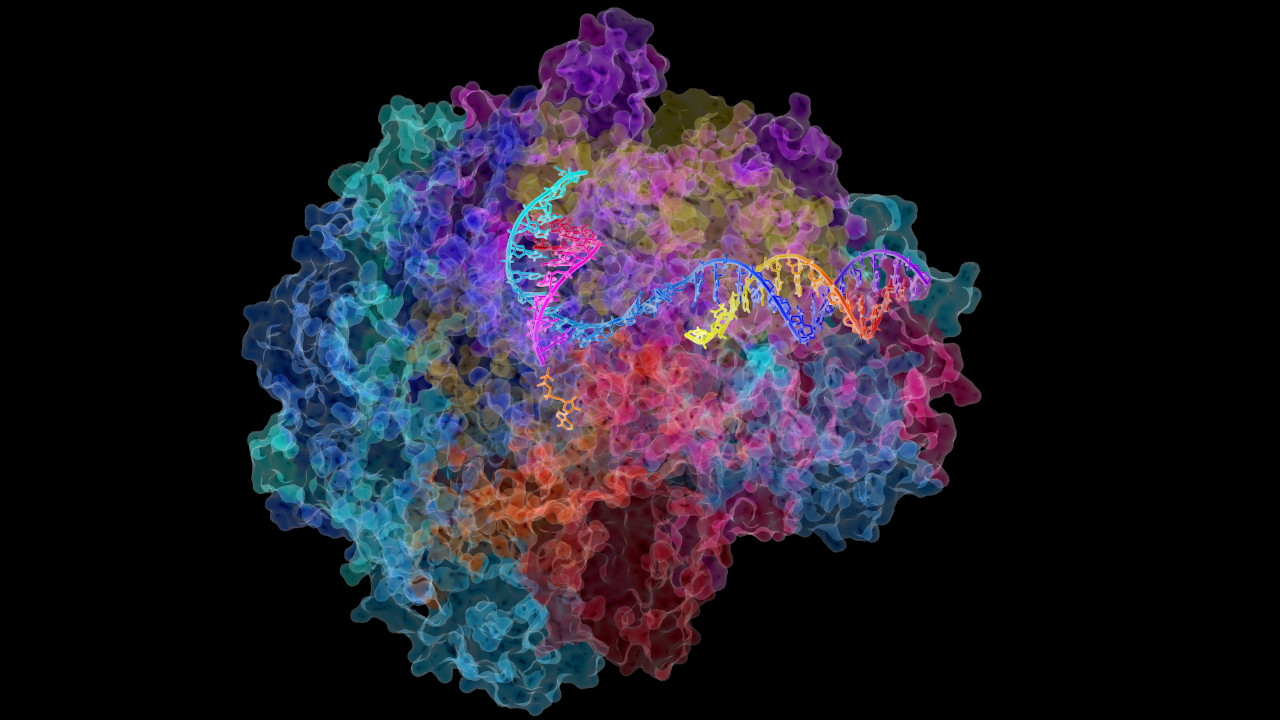
In the production of special chemicals and pharmaceuticals, enzymes offer the advantage that they can produce far more complex compounds than chemical syntheses and only produce the levorotatory or dextrorotatory form of a molecule in each case. This is significant because chiral molecules can act very differently biologically even though they are mirror images of each other. Examples of enzymes for single-step reactions are transaminases and ketoreductases, which are used to produce drugs such as esomeprazole for stomach ulcers, montelukast for respiratory diseases or the anti-diabetic drug sitagliptin.
Page 4 of 5
4) Cell-free enzyme cascades - in several steps to the product
The majority of today's chemical products cannot be produced in simple one-step syntheses from suitable starting materials. Usually, several synthesis steps are required to synthesise the final product. Classically, the individual synthesis steps are carried out sequentially and with purification of the intermediate products. This approach is economically and ecologically inefficient.
Cell-free enzymatic multi-step reactions - also known as enzyme cascades - have enormous potential here. They string together several enzymatic catalysis steps, which in the end enables a highly efficient, highly specific process to produce chemically highly complex molecules.
Today, it is already possible to realise complete metabolic pathways up to the products ethanol, isobutanol and butanol as an enzyme cascade in a reaction vessel. The advantage of cell-free enzyme cascades becomes clear in glycolysis, a ten-step process that produces pyruvate from glucose. Unlike the natural process in cells, the cell-free enzyme cascade can be operated at 50 to 60 degrees Celsius, which leads to higher reaction rates and thus better productivity. By combining enzymes that do not occur together in nature, such cascades can also be simplified compared to natural reaction pathways. Artificial glycolysis, for example, requires only four enzymes instead of ten for the reaction described. In this way, costs can be significantly reduced.
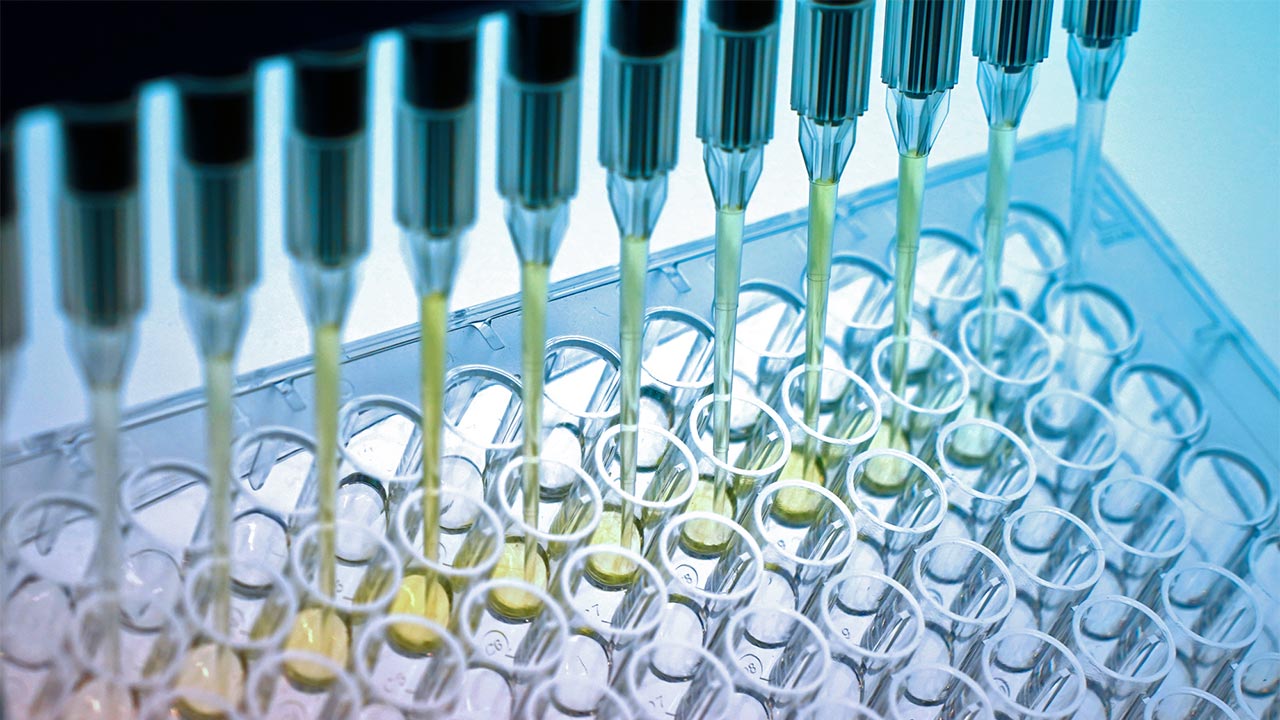
The possibilities for multi-step reactions are manifold. For example, researchers led by Tobias Erb have developed the CETCH cycle, a 17-step enzyme cascade that uses the greenhouse gas carbon dioxide as a substrate and produces the valuable substance malate from it. The Marburg researchers have also developed a redox enzyme that is one of the most efficient CO2-converting biocatalysts in the world. Researchers in the Fraunhofer Max Planck project eBioCO2n are taking advantage of this. They have designed a synthetic enzyme cascade with which electricity-driven CO2 can be used to produce special chemicals.
In 2021, a Chinese research group also caused a stir by developing a metabolic pathway consisting of eleven reaction steps called ASAP, which can be used to synthesise starch from carbon dioxide. In plants, this requires about 60 steps. A team led by Volker Sieber has developed a kind of artificial photosynthesis for the production of amino acids from CO2. CO2 is processed into methanol using green electricity and hydrogen. Synthetic enzymes then convert this intermediate product into the amino acid L-alanine very effectively and in high yield in a multi-stage process. Tobias Erb's team has in turn constructed an artificial metabolic pathway in which the intermediate product formic acid plays an important role.
This explanatory video also looks at cell-free processes in sustainable biorefineries
Rational design
Today, experts usually design new enzyme cascades according to the principle of rational design and the approach of synthetic biology. Starting from the target molecule, they mentally divide the reaction chain into subunits. Then they combine enzymatic reactions and sometimes chemical syntheses to correctly assemble the individual steps.
By immobilising the enzymes on a carrier material - the so-called matrix - researchers want to bring the biocatalysts close together so that the product of one enzyme ends up directly with the next enzyme for which it is the substrate. In this way, reactions could take place particularly efficiently.
A central challenge is to actually transfer these concepts into industrial application. The reaction conditions must be selected in such a way that all the enzymes involved in a cascade can be sufficiently active.
Cell-free multi-step reactions are used commercially, for example at Eversyn in Marburg, to produce nucleotide sugars and oligosaccharides.
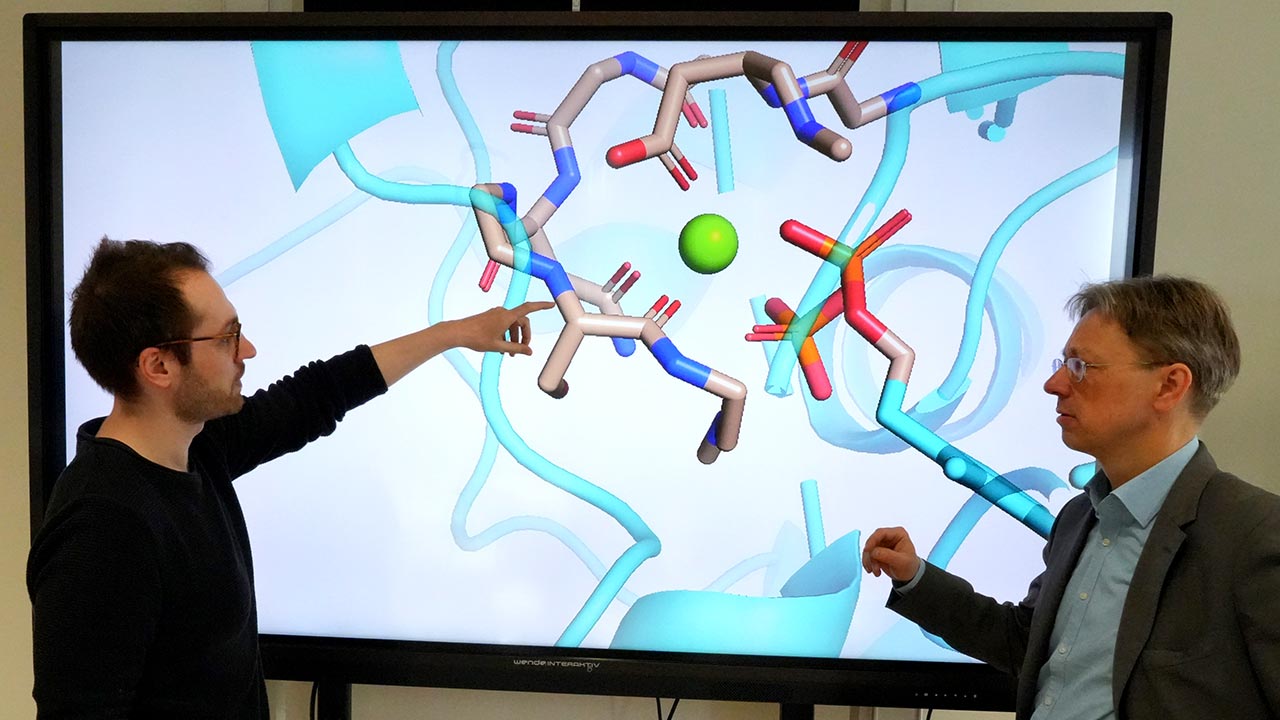
Page 5 of 5
5) Outlook: Combining smart approaches
Innovative processes and technologies in biochemistry and molecular biology have raised the hopes of many researchers and companies to develop even more effective approaches for cell-free protein synthesis and enzyme processes.
AI-based enzyme engineering
For example, there has been great progress in modelling enzymes to draw conclusions about how their building blocks need to be changed to achieve certain properties. This can save an enormous amount of development time. Artificial intelligence is an important tool here. Modern IT methods also help to better understand the molecular dynamics in the active centres and to optimise the interaction of enzyme and substrate. Many modern approaches are still in development - from rational design to enzyme engineering.
Regenerating cofactors and energy carriers
In order to achieve the highest possible product yield in a biotech process, the enzymes often need the appropriate cofactors. These small, organic molecules are consumed in the course of each individual reaction and must be regenerated to be ready for use again. Providing them again in large quantities is very expensive and thus uneconomical for industry. Therefore, concepts for cofactor regeneration are being developed in the biotechnology sector. The energy supply of cell-free systems can also be solved by regenerative concepts. In addition, cofactors are very unstable, so more robust alternatives are being worked on.
Cell-free bioproduction in BMBF funding
Projects on cell-free bioproduction have been and are being worked on in the following BMBF funding measures:
• Basic technologies for the next generation of biotechnological processes (Biotechnology 2020+)
• Future technologies for the industrial bioeconomy: focus on biohybrid technologies
• Tailor-made biobased ingredients for a competitive bioeconomy
Smart bioreactors with fluid dynamics
For a long time now, experts have not only been looking at individual biocatalyst molecules, but also at multi-enzyme complexes in which multi-step reactions could take place in a confined space. Also of interest are fusion proteins, in which genetic modifications cause two proteins to lie together. Their active centres are thus close together, which is favourable for the cofactor in redox reactions, but also generally improves the reaction speed. The geometries of matrices and reactors are also constantly being optimised to improve process efficiency. In terms of process technology, it is also difficult to scale up the conditions for enzyme cascades developed on a laboratory scale to industrially relevant dimensions.
One hope here rests on so-called flow chemistry, a continuous flow technique in miniaturised fluidised bed reactors. Immobilised enzymes exist here in different compartments that can be optimally coupled with each other. ContiVir, a spin-off of the Max Planck Institute for Dynamics of Complex Technical Systems in Magdeburg, is currently developing such a reactor to produce active substances for viral gene therapy.
In many cases, cell-free systems are still in the conception and testing phase. The biggest challenge is the step into industrial application. Researchers are concentrating here on reducing costs and developing long-lasting and particularly high-yield production systems. There is also great potential in the intelligent combination of in vitro systems and cell-based systems, for example for testing synthetic metabolic pathways in synthetic biology.