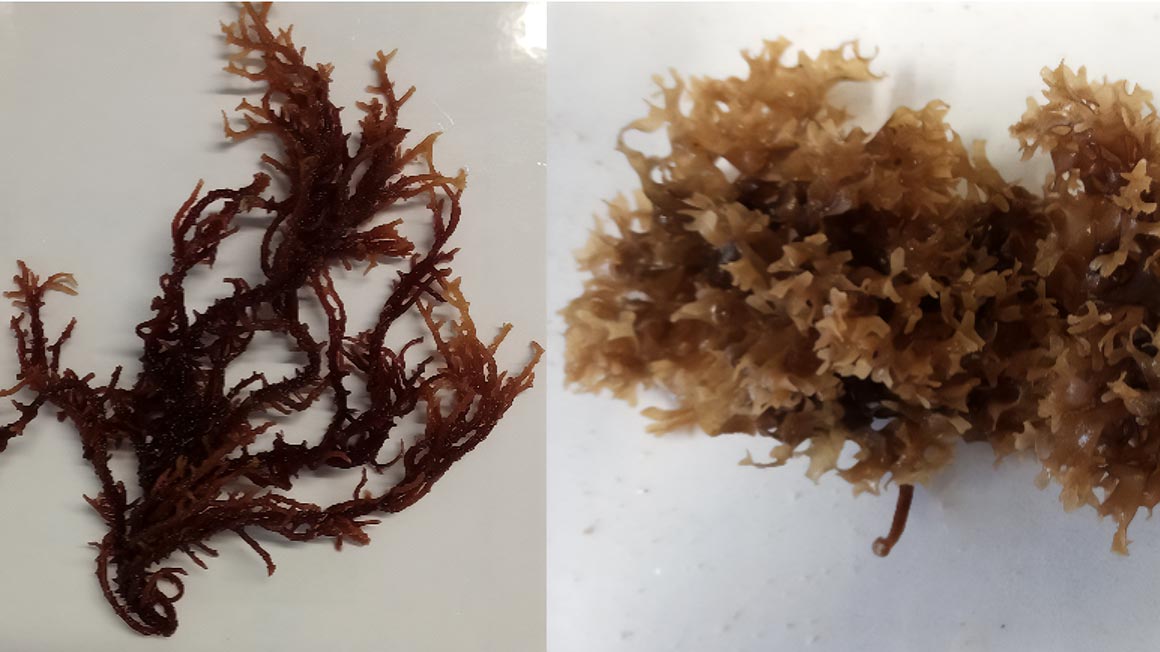
Carrageenan – it sounds exotic, but for many people, it’s already a part of everyday life. In yogurt, ice cream, or toothpaste, carrageenan helps ensure the right consistency and stability. The plant-based gelling and thickening agent is commonly used in foods as additive E 407 and is approved without quantity restrictions. The substance comes from red algae and consists of long sugar chains with many so-called sulfate groups. These very sulfations make carrageenan both intriguing and complex. Its properties change drastically depending on how many sulfate groups there are and where they’re located. This holds huge potential, but until now, has been difficult to control in a targeted way.
New variations of an established additive
“I worked for a long time in industry with biopolymers from red algae,” says Volker Sieber, a biotechnologist at the Technical University of Munich. “That’s why I was excited by the idea of producing variations of these polymers with different properties.” The result was the BioTrim research project.
The goal of the researchers in the BioTrim project was to enable tailored sulfation. The team aimed to deliberately modify the structure and function of carrageenans, paving the way for new applications in the food, cosmetics, and biotechnology sectors. They were also interested in one particular sugar component found in carrageenan: 3,6-anhydro-D-galactose, a promising candidate for bio-based chemicals.
Searching for enzymes in marine bacteria and databases
In samples containing marine bacteria, the researchers specifically searched for enzymes that can break down carrageenan, known as sulfatases. “At the same time, we searched databases for similar enzymes that might do what we needed,” explains Sieber. Ultimately, this turned out to be the faster approach. Using bioinformatics methods, the researchers identified specific gene clusters in bacterial genomes that pointed to these types of enzymes. They then produced the most promising candidates in E. coli, a model bacterium widely used in biotechnology, and tested their effectiveness with a custom-developed assay.
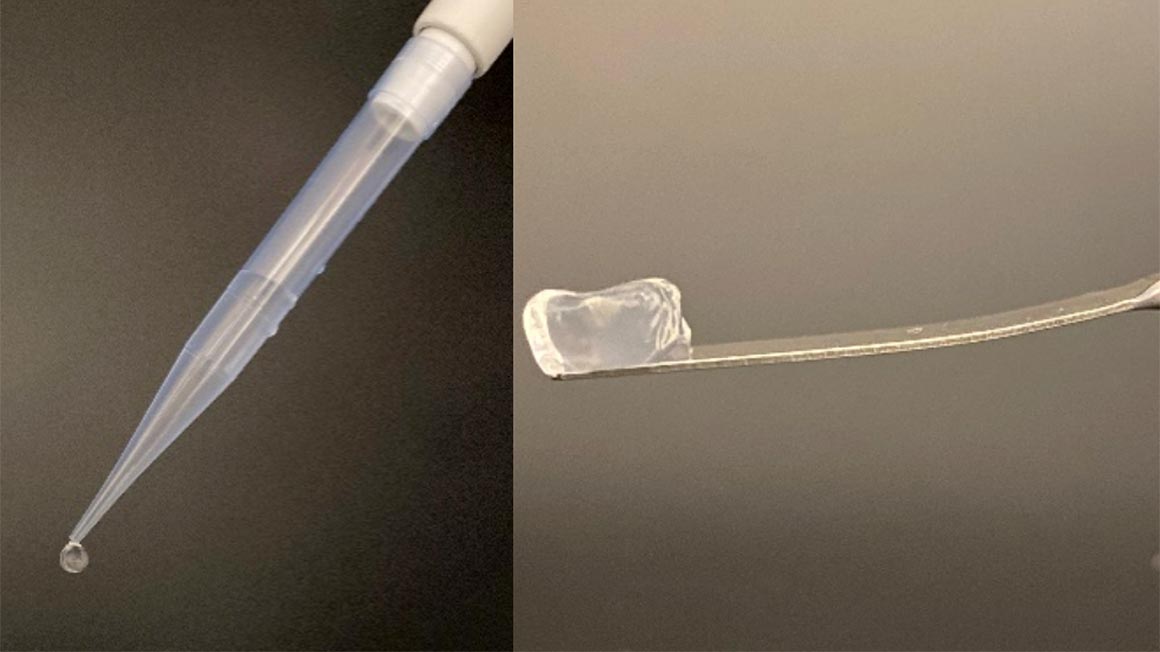
Several promising enzymes identified
The researchers identified several novel sulfatases, including some with an ability to remove sulfate groups from very specific positions that had been previously unheard of. One particularly exciting discovery was that one of these enzymes could convert gel-forming κ-carrageenan into a new form that created significantly stronger gels, similar to those made from the much more expensive agarose. Conversely, ι-carrageenan was transformed into a structure with nearly no gelling ability at all – even though the molecules differed by just one sulfate group per subunit. “It was amazing to see, when we added the polymers to a solution, how the enzymes turned them into a gel,” Sieber recalls.
The team also succeeded for the first time in developing a cell-free biotechnological process to extract 3,6-anhydro-D-galactose from carrageenan. The current yield is around 13% for κ-carrageenan and 7% for ι-carrageenan – a promising start that still requires optimization. A key step in this targeted transformation was combining various enzymes: first, a hydrolase was used to break the carrageenan into smaller fragments. Then, sulfatases were applied to selectively remove sulfate groups. Finally, additional enzymes broke the sugar chains down into their individual components – including the sought-after sugar building block 3,6-anhydro-D-galactose.
The BioTrim project
The BioTrim project ran from February 2020 to January 2024 and received approximately €340,000 in funding from the German Federal Ministry of Education and Research under the “Bioeconomy International” program. In addition to the Technical University of Munich, the Universidade Estadual Paulista in Brazil was involved.
Potential for the food and cosmetics industries
The project’s work opens new doors: with custom-designed enzymes, carrageenans can now be modified to meet specific needs – for example, to create tailored textures in food or bioactive ingredients in cosmetics. “In food technology, you could achieve the same effects using smaller amounts of carrageenan as an additive,” says Sieber. At the same time, this approach creates a sustainable path to valuable platform chemicals from marine resources – without relying on fossil raw materials.
The project lead is highly satisfied with the outcome: “At the beginning, we didn’t know whether we’d find enzymes that would even work on polymers,” he recalls. Polymers are challenging substrates for enzymes. “So, discovering strains with the desired activity was a real highlight.” In the end, the team accomplished what they set out to do.
Next, the researchers plan to further optimize the enzymes, explore their potential uses, and advance the production of bioactive oligosaccharides. They are particularly focused on developing new carrageenan structures with specialized functions and improving process efficiency.
Author: Björn Lohmann