What is precision fermentation?
Microorganisms are the heroes of fermentation: they are at work in sourdough, yogurt or in the steel tanks of the biotechnology industry. The cells initiate material conversion processes and produce interesting products through fermentation or other metabolic processes. Thousands of years ago, people used microorganisms to make food more durable, digestible and nutritious. Without them we would not have beer, wine, bread or cheese.
The fact that microorganisms are at work here was not discovered until much later. A few decades ago, industrial biotechnology then began to systematically optimize these natural processes in order to manufacture sought-after products more efficiently. Today, bacteria, yeasts, molds and other cells can be converted with increasing precision and used for the industrial production of certain substances.
For a long time, biotechnology has primarily used cells and microorganisms to manufacture products that they naturally form as part of their metabolism. The primary goal of research was then to further increase the proportion of these compounds, to establish more favorable substrates as raw materials, or to optimize the process conditions and purification steps in such a way that the yield became higher.
Since the 1980s, genetic engineering methods have been used to modify the metabolism of production organisms so that they produce desired products in large quantities and prevent the formation of undesired by-products. Since then, the toolbox of industrial biotechnology has expanded greatly: thanks to advanced molecular tools and information technology processes, industrial biotechnology today is about to open up a new level of possibilities.
To produce a desired biological molecule from a defined raw material, researchers use synthetic biology, genome editing, artificial intelligence and big data to design microorganisms, animal and plant cells in such a way that they produce complex biological products with high efficiency. Microorganisms or other cells are thus converted into cell factories and equipped with the appropriate genetic programming. The bioprocess conditions, purification steps and other process engineering tasks must also be tailored to ultimately enable an optimal result.
In recent years, a new term has emerged for this: Precision fermentation. Because it is a successive development of industrial biotechnology, the term precision fermentation has so far neither been uniformly defined nor established everywhere. Linguistically, the English term precision fermentation is in line with established buzzwords such as precision medicine, precision engineering and precision biology. However, the common denominator of all definitions of precision fermentation is consistently that microorganisms or cells are programmed and cultured to produce almost any complex organic molecule. It is thus a holistic approach that combines state-of-the-art methods from biology and information technology.
Innovations have opened up groundbreaking opportunities for biotechnology in a variety of disciplines. However, they are often associated with high initial costs. Therefore, precision fermentation focuses on products with high value, for example pharmaceutical compounds or value-added products in the food sector.
The more established the individual methods become, the more the costs fall. As a result, platform chemicals, materials and energy sources are also becoming the focus of research and development. Last but not least, the industry's move away from petrochemical processes to protect the climate is driving precision fermentation forward. As a raw material for supplying cells or microorganisms, the processes therefore prefer to rely on biogenic residual and waste streams so as not to compete with biomass for food and feed.
However, the use of biomass as a resource depends, among other things, on efficient processes in order to be sustainable. Precision fermentation not only has ecological and medium-term economic advantages. It also makes it possible to endow the molecules to be produced with new functions or more specific modes of action.
Page 2 of 4
Here's what players from the biotechnology industry have to say
For many it is industrial biotechnology of the latest generation, for some it is merely a trendy term for well-known technologies such as metabolic engineering or approaches such as synthetic biology. The term precision fermentation has not yet been clearly defined. That's why bioökonomie.de asked players in the German biotechnology scene what the term precision fermentation means to them:
Dr. Marc Struhalla (Managing Director of the Leipzig-based enzyme manufacturer c-LECta):
„We like the term because it makes the connection to the production process. From our point of view, there are many technological innovations, but they often do not translate into scalable, efficient production processes. But that's what it ultimately comes down to. Industrial biotechnology is about production process innovations. That's why, in our view, the term 'precision fermentation' has its raison d'être.“
Prof. Christoph Wittmann (Institute for Systems Biotechnology, Saarland University):
„For me, precision fermentation integrates custom cell factories with tailored bioprocesses - both molecular and technical elements combining the disciplines of genomics, systems biology, synthetic biology, metabolic engineering, advanced bioprocess technology and digitization. It's a holistic approach that also implies resource-efficient, selective product manufacturing.“
Sam Tadman (Material Innovation Analyst, start-up Cambrium, Berlin):
„The term precision fermentation is used by some people to refer to the use of modern biotechnological tools to rationally re-engineer microorganisms to produce a specific molecule through genetic modification. We ourselves do not use the term, since the fermentation process itself does not change. Instead, we prefer to talk about the particular technologies used in each step.“
Christian Poppe (Director Global Public Affairs & Sustainability, start-up Formo):
„The term clarifies that it is a technological evolution of fermentation, which we have known for centuries in food production. It thus combines innovation and tradition in one term. Precision fermentation stands for innovative, sustainable food production in a wide variety of applications in the global food system, including dairy. It represents the opportunity to leverage new potentials of biotechnology to feed a growing world population while effectively contributing to the fight against global warming.“
Page 3 of 4
Tools and methods
The latest generation of industrial biotechnology uses methods from bioanalytics, molecular biology, systems biology and bioprocess technology. These methods and processes are increasingly being digitized and networked.
Bioanalytics
Genome analysis refers to all methods used to decipher and analyze the genetic material of an organism. This includes both the determination of the sequence of the nucleotide building blocks of DNA - sequence analysis - and the investigation of what this sequence does biochemically for the organism. This functional genome analysis includes transcriptomics and proteomics, which analyze how the activity of the gene is regulated and which proteins are produced under which conditions.
Omics technologies refer to machine-supported high-throughput methods that can be used to analyze biomolecules such as DNA, RNA, proteins or polysaccharides in their entirety. Omics technologies continue to improve and today provide spatially and temporally resolved information in huge numbers, which is evaluated by self-learning, bioinformatics methods.
An important trend is the miniaturization and automation of systems. Here, so-called microsystems in particular hold great potential. One application that is currently revolutionizing the life sciences is single-cell analysis using microfluidic chips. The latest generation of sequencing techniques makes it possible to capture even small amounts of genetic material or proteins from individual cells. This makes it possible to measure which genes are active in a cell at any given time. This enables researchers to determine the molecular profile of a cell.
In single-cell bioreactors, bacterial cells can be systematically examined with regard to their growth and selected metabolic processes. Miniaturized screening systems are also increasingly used for strain development in industrial biotechnology.
BMBF brochure: The Tools of the Bioeconomy (in German)
The BMBF brochure The Tools of the Bioeconomy presents many of the technologies and processes mentioned in this dossier in illustrated short profiles.
PDF Download
Molecular biology tools
Classical genetic engineering: Genetic engineering methods are used to introduce genetic material into the genome of living organisms. This can be a foreign DNA section from other organisms, or it can originate from the same species. After gene transfer, the genetic material is inserted into the genome at a random location and read. Genetic engineering methods can be used to introduce the modified molecular blueprint of a desired molecule - such as an enzyme - into other bacteria. In this way, the bacteria are programmed to produce the desired enzyme in high yield and purity.
Genome editing works in a much more targeted way than classical genetics. Thanks to programmable gene scissors, DNA segments can be precisely cut out of the genome of living cells and inserted into them. In this way, genes can be switched off and on, inserted or removed. Currently, the best-known gene scissors are CRISPR-Cas.
Metabolic Engineering: When complete metabolic pathways in a microorganism are specifically altered or redesigned, experts refer to this as metabolic engineering. In the past, the main aim was to increase the production volume of the target product. To do this, research teams eliminated by-products or downstream products, for example, or taught the organism to utilize less expensive raw materials as substrates. However, particularly in the context of precision fermentation, researchers are increasingly pursuing the goal of establishing entire biosynthetic pathways anew in an organism, either designing them from scratch or transferring them from other organisms. For example, the anticancer drug Taxol has so far been produced by yew trees - conifers that, with their slow growth, also have low production rates. The aim is therefore to transfer this biosynthetic pathway to the established production organism baker's yeast.
System-oriented approaches
Synthetic biology: The aim of this interdisciplinary approach is to design and construct biological systems in a modular fashion. In this way, biological components, cells or organisms are to be equipped with properties and capabilities that do not occur in nature in this way. This offers great opportunities for researching specific processes in simplified cells. But it also makes it possible to create cell factories in which there are no undesirable side effects on the biosynthetic pathway from the outset and in which everything is optimized for the production of the target product.
Systems biology has a similarly holistic approach to synthetic biology - except that the aim of this interdisciplinary research approach is to analyze dynamic life processes and complex biochemical networks in their entirety and to describe them with the aid of computer-aided mathematical models.
So far, for example, it has been possible to better understand signaling networks or metabolic networks in microorganisms and to optimize the microorganisms for industrial production processes. For example, by predicting optimal material flows or high product yields.
Bioprocessing
Bioprocess technology is the process engineering part of precision fermentation. It comes into play at three stages: in the design of the actual process and the bioreactor, in process monitoring and control, and in purification. Challenges in process design are many: Cells must be supplied with nutrients and oxygen without agitators damaging the cells. Growing biofilms can disrupt parts of the system. Continuous processes are economically attractive, but technically difficult and require more effort to retain cells or purify the product. However, only optimal process parameters enable optimal product yields - and these are the goal of precision fermentation. This is not a fundamentally new goal in biotechnology, but advances in sensor technology and real-time process control enable further efficiency gains.
In addition to modeling the smallest unit in the bioprocess - the cell - modeling the surrounding processes and their optimization is becoming increasingly important. Thus, a description of the conditions in the bioreactor with regard to oxygen saturation or substrate availability is possible. Models have now also been established for the subsequent purification steps, by means of which the best conditions for product purification can be determined with significantly fewer experiments. Process modeling tools have the potential to significantly reduce development costs, make the process chain more favorable and the utilization more flexible.
Digitization
Digitalization makes three main contributions: Artificial Intelligence (AI), sensor technology and real-time process control. Methods of AI, in particular its strength of pattern recognition, make it possible to identify connections between the genome or proteome and characteristics of an organism that would otherwise remain invisible. In addition, AI enables targeted optimization of the structure of enzymes, allowing them to perform their task particularly efficiently and form the target product in high concentrations.
Sensor technology comes into play on the one hand when large quantities of experimental results need to be evaluated automatically, for example in the search for those cells in an experiment that produce a product in the highest concentration. Experts refer to this process as high-throughput screening. On the other hand, only sophisticated sensor technology enables precise real-time monitoring of bioprocesses and constant adjustment of process parameters to the optimum. This, too, is not specific to precision fermentation, but it contributes to its success.
Page 4 of 4
Examples of applications
Precision fermentation can be used for products in many industries. In the following, some examples illustrate this potential with manufacturers from Germany.
Astaxanthin: dye from the cell factory
Astaxanthin is used in fish farming as a feed additive, where it strengthens the animals' immune defenses and gives farmed salmon its reddish color, for example. The cosmetics industry uses astaxanthin because of its antioxidant effect: it protects the skin better from UV radiation than vitamin E does. For a long time, the green alga Haematococcus pluvialis was the only bio-based alternative source to the petroleum-based synthesis of astaxanthin. Corynebacterium glutamicum, which is industrially established as a cell factory, naturally produces astaxanthin, a dye from the carotenoid group. The start-up Biocomer is in the process of optimizing the fermentation of astaxanthin by C. glutamicum and developing it to market maturity. It has already received several awards for this approach.
Isobutene
The French company Global Bioenergies operates a demonstration plant in Leuna for the production of bio-isobutene from wheat straw. The plant can produce 100 metric tons per year with the help of bacteria. To this end, the company has used synthetic biology methods to modify the bacterium Escherichia coli so that it possesses the necessary enzymes to produce isobutene - because no such metabolic pathways exist naturally in any known bacterial strain. Until now, the hydrocarbon isobutene has been obtained exclusively from crude oil. Annual global production is worth around 25 billion U.S. dollars.
Vegan cheese substitute
There are several plant-based cheese alternatives. However, Formo, a young company based in Berlin, produces a variant made from real milk protein (casein) and whey protein. Neither comes from animal sources, but is fermented by yeast. The company has biotechnologically integrated the synthesis pathways of these proteins into the yeast cells. Together with vegetable fats and carbohydrates, this creates a vegan cheese substitute. According to the company, it will not contain cholesterol or allergens. The first animal-free cheese products are expected to hit the market in 2023 and be on par with conventional products in terms of price by 2025. The first variety could be a mozzarella, with other cheeses and dairy products to follow later.
Spider silk protein
Spider silk is a fascinating natural product because it combines an antibacterial effect and extremely high stability. However, natural extraction is limited or expensive for obvious reasons. The artificial production of spider silk has therefore enabled a wide range of applications. The pioneer in this field is the Martinsried-based company AMSilk, once a spin-off of the Technical University of Munich. AMSilk produces the spider silk protein in the bacterium Escherichia coli, has scaled up this bioprocess and has also developed a special process to spin fibers from the protein solution. The spider silk proteins can now be found in skin care products and nail polish, in composite materials for aircraft wings, as a coating for medical implants, or in textile applications in sports shoes and car door handles.
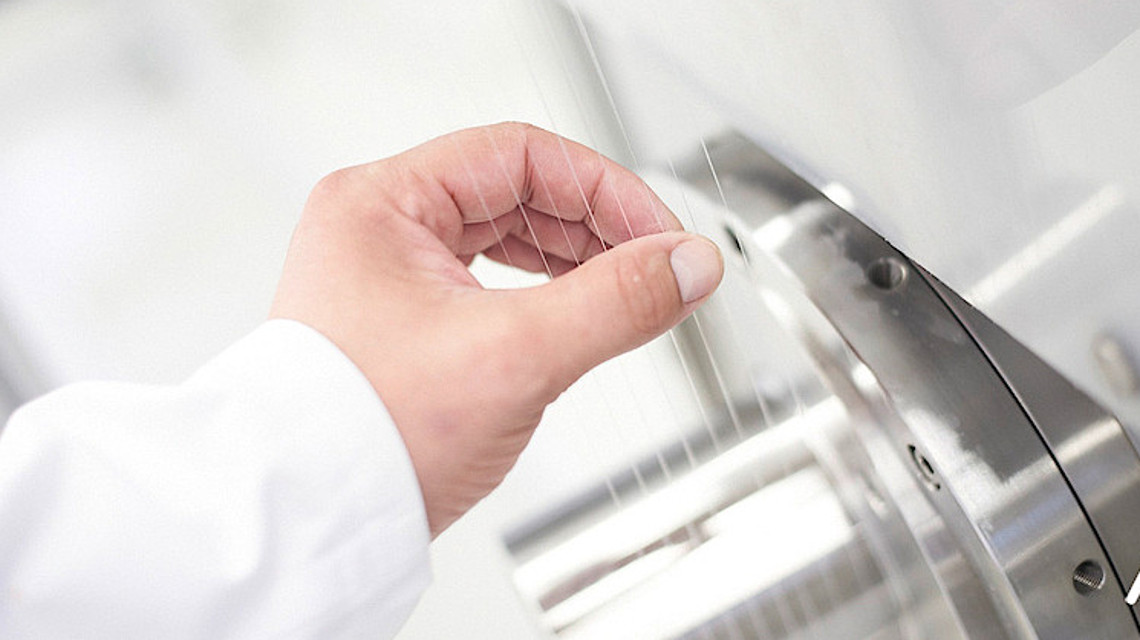
Vegan collagen
Collagen is contained in cosmetic products. It protects and moisturizes the skin and makes it more elastic. Until now, collagen has been obtained from animal bones and skins. The Berlin-based start-up Cambrium uses fermentation to produce a nature-identical collagen for the cosmetics industry. The aim is to further improve the functionality of the collagen compared to its natural counterpart. To this end, Cambrium relies on a technology platform that combines protein engineering, machine learning and laboratory automation. In the future, this platform will also be used and adapted to produce special proteins for other industries.
Further examples from the US
Hemoglobins are a group of substances that play an important role in the typical taste of meat. They are a component of animal hemoglobin, which colors blood red, and whose plant equivalent is leghemoglobin. The US company Impossible Foods produces this dye in genetically modified yeast cells as an ingredient for plant-based burger patties.
The US company Nature's Fynd produces protein in mushrooms of the species Fusarium flavolapis for meat substitutes and milk substitutes. The protein, named Fy, contains all nine essential amino acids.
The EVERY Company (formerly: Clara Foods) has genetically modified yeasts so that the cells form chicken egg white. The animal-free protein can replace chicken egg white wherever it is already used in food production. Many companies rely on such "drop-in" solutions.
The US company Jellatech produces collagen and gelatin in a bioreactor. To do this, it uses skin cells from animals, which are then multiplied in a biotechnological process. The collagen formed by the cells is extracted and purified.