Hydrogen - a key element of the energy transition
Hydrogen is an energy carrier that can be produced with low CO2 emissions. The light gas has a much higher energy density than conventional fuels. Its combustion produces water and heat - so its use creates no carbon footprint.
That's why hydrogen is seen as a key element of the energy transition: In the future, hydrogen will power climate-friendly airplanes, ships and trucks, or make the production of green steel possible. For hydrogen to be called "green," the gas must be produced by electrolysis of water with electricity from renewable sources - a process that is energy-intensive and costly.
One promising alternative is biobased hydrogen, which is produced from biomass or with the help of microorganisms and algae. This dossier analyzes which biobased production methods are available for hydrogen generation and what the potential and technical development status are for the various areas of use.
Production routes and their colors
Hydrogen is colorful - at least in its linguistic classification: Gray hydrogen dominates the market today and comes from fossil sources. It is mostly produced from natural gas, a process in which about ten tons of carbon dioxide (CO2) escape into the atmosphere for every ton of hydrogen, and which takes place at temperatures of 500 to 1,000 degrees Celsius. Besides chemical production from natural gas, hydrogen is now mainly produced as a by-product of chemical processes. If CO2 is captured and stored during production, this is referred to as blue hydrogen. Blue hydrogen thus continues to use fossil resources and does not help with the energy transition. Turquoise hydrogen is produced from methane that is thermally cracked. This is CO2-neutral if the thermal energy comes from renewable sources and the resulting solid carbon is permanently bound instead of being released into the atmosphere as CO2. Only green hydrogen is guaranteed CO2-neutral. It comes from renewable sources, such as the electrolysis of water powered by electricity from renewable sources or biological resources.
Bio-based hydrogen is either obtained from biomass or produced by means of living biomass - i.e., through the metabolic activities of living organisms.
Fuel cells and energy storage
One ton of hydrogen contains 33,330 kilowatt hours of energy. It can be used directly, processed into methane or mixed with natural gas as fuel. Fuel cells are used to convert the energy into electricity. In them, hydrogen and oxygen react with each other, producing water and electricity. If the resulting process heat is used, this is known as cogeneration.
Fuel cells with combined heat and power are used as heating in larger buildings, for example. While several hundred thousand buildings in Japan are already heated in this way, the combination of heat pump and photovoltaics is more popular in Germany. Fuel cells also power vehicles, from forklifts to buses. Prototypes and small series exist in aviation, shipping and train transport. Worldwide, however, the number of fuel cells in use is only in the five-digit range. The importance of hydrogen as an energy storage medium is currently being intensively investigated. For example, surplus wind power could be converted into hydrogen, which could then be fed into the natural gas grid or used in the mobility sector as a fuel or raw material for the production of synthetic fuels (e-fuels).
Hydrogen is also an indispensable reducing agent in the chemical process industry, with the help of which carbon atoms are converted into hydrocarbons. In an industry that is increasingly relying on renewable carbon sources (for example CO2) and striving for a closed technical carbon cycle, green hydrogen is playing an increasingly important role.
National hydrogen strategy
So far, green hydrogen has not been available in sufficient quantities to make a significant contribution to the energy transition. This is to change according to the plans of the German government, which adopted the National Hydrogen Strategy in 2020. The aim is to strengthen and drive the market ramp-up nationally and internationally by 2030. The new German government also wants to quickly accelerate the development of a hydrogen industry and has announced a revision of the hydrogen strategy to this end. But Germany alone will not be able to produce enough hydrogen. Researchers at Jülich have calculated a demand of around 414 terawatt hours for the year 2045, almost half of which will have to be imported.
The focus is to be on green hydrogen, although the German government assumes that blue and turquoise hydrogen will also play a role initially and above all internationally. The National Hydrogen Strategy mentions biobased hydrogen only at one point - as forward-looking basic research. This dossier presents different biobased approaches.
Page 2 of 7
Biomass gasification and biogas reforming
Hydrogen is currently produced in industry mainly by the conversion of fossil hydrocarbon compounds. To make production more sustainable and essentially CO2-neutral, biomass can be used instead of fossil organic compounds. Wood, agricultural residues such as straw, or municipal waste can be used for this purpose. What they have in common is that, as resources, they do not compete with food production.
A biobased process that can produce hydrogen via a thermochemical route is biomass gasification. For this, the biomass must first be pretreated. It is important to reduce the moisture content of the biomass to below 15 percent by volume. After drying, the biomass can be fed into a gasifier reactor. Wood components such as the celluloses, hemicelluloses and lignin decompose to form coke and volatiles. The coke is then brought into contact with water vapor and oxygen at temperatures of about 1,000 degrees Celsius, converting it into a gaseous energy carrier. However, the hydrogen yields are lower compared with established processes based on fossil raw materials.
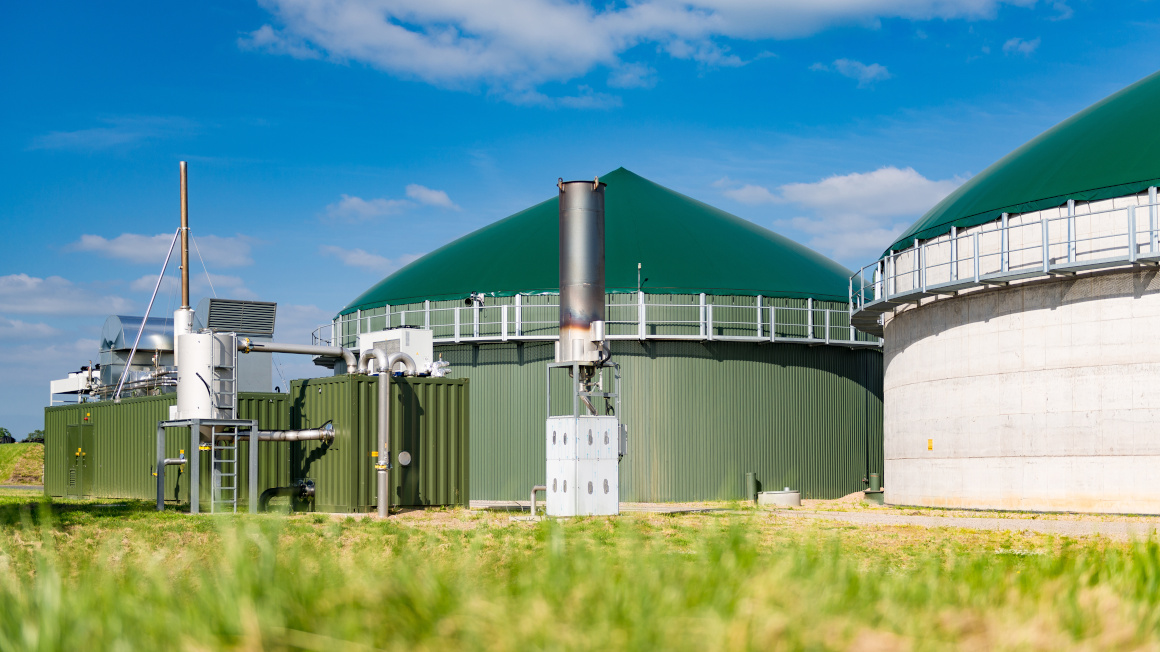
Steam reforming of biogas to produce hydrogen is based on technologies known from natural gas reforming. In this process, methane is reacted with water to form hydrogen and carbon monoxide (CO). In combination with a downstream water gas shift reaction, the carbon monoxide produced can be converted with water to CO2 and further hydrogen.
The actual reforming step usually takes place at temperatures above 800 degrees Celsius and with the aid of a nickel catalyst. These catalysts require a sulfur-free gas, which is why prior processing steps for coarse and deep desulfurization are necessary. The CO content in the reformate is then reduced by a so-called water gas shift stage and the hydrogen is separated.
Page 3 of 7
Biophotolysis: Photosynthesis to hydrogen
Green hydrogen is usually produced by electrolysis: Here, water is split into its components oxygen and hydrogen with the help of electricity. In photolysis, on the other hand, the water is split with the help of light energy.
This reaction underlies the central metabolic process on earth: photosynthesis. From sunlight and the energy-poor inorganic substances CO2 and water, plants build the energy-rich organic compound glucose. In light reaction, a light-driven partial process of photosynthesis, water is split. With the addition of light energy, catalyzed by enzymes, the water molecules are split into oxygen, protons (i.e. hydrogen ions) and electrons.
If one succeeded in producing hydrogen by photosynthesis processes, one could fall back on the arguably most abundant resources on earth: Water and sunlight.
Biophotolysis includes all biological processes for the production of hydrogen by photosynthesis. Photosynthetic microorganisms can produce hydrogen directly from sunlight. The hydrogen is a by-product of the microorganisms' metabolism. Cyanobacteria as well as some green algae (Chlorophyceae) are able to produce hydrogen from water by photolysis. Here, the hydrogen often serves as a kind of valve to reduce excess protons produced during high photosynthetic output and to discharge them out of the cell.
Direct and indirect route
The green alga Clamydomonas reinhardtii is one of the best hydrogen producers known to date. In the so-called direct biophotolysis, the unicellular alga produces hydrogen (H2) from water and sunlight under anaerobic conditions - i.e. in the absence of oxygen - as well as of sulfur. In the first step, the water is split into hydrogen ions and oxygen at photosystem II and the photosynthetic electron transport chain is set in motion. This is where the so-called hydrogenases come into play as key enzymes. They catalyze the simple reaction of protons and electrons to molecular hydrogen.
The only byproduct is oxygen. Since only water, sunlight and CO2 are required as starting materials, this method of generating green hydrogen is considered to be particularly sustainable. The advantages include that the processes take place under normal ambient conditions and are therefore easy to implement in terms of process technology, and that the microorganisms (and thus their enzymes as biocatalysts) reproduce themselves in the bioreactor. However, there are still a number of challenges to be overcome for efficient production: For example, the microorganisms must still receive sufficient sunlight even at high culture density, and the enzymes involved are inhibited by the oxygen produced.
In the absence of oxygen, some cyanobacteria and green algae operate an alternative metabolic pathway called indirect biophotolysis. Here, in a first step, the microorganisms produce carbohydrates and store them. If a lack of oxygen occurs again, the cell stops certain processes and can use the electron currents thus released to form hydrogen through the enzyme hydrogenase. The microalgae chlorella masters this process particularly effectively. Because industrially interesting lipids are produced at the same time, this method of synthesizing green hydrogen has the potential to become economically viable in the long term. However, CO2 is also produced in the process, and the actual hydrogen yield has so far been low.
So far, it has not been possible to produce hydrogen on a large scale using photobiological processes, and no commercially relevant scale-up exists yet for biophotolysis. One problem is the oxygen sensitivity of the microorganisms involved, since oxygen is also produced in large quantities by water splitting. Currently, the efficiencies are therefore rather low, so that only 1.5 % of the irradiated solar energy can be converted to hydrogen. Immediate removal of the oxygen produced could increase the efficiency to 3-10 %. In view of the technical development status, a study by the University of Erlangen-Nuremberg gives a Technology Readiness Level (TRL) of 3 for this process, i.e. experimental proof of the concept at best.
Biotechnological processes
According to experts, large-scale hydrogen production will only be possible through biotechnological modification of the microorganisms used.
To produce solar hydrogen, researchers led by Kirstin Gutekunst at the University of Kassel are focusing on a targeted modification of the hydrogen metabolism in cyanobacteria. Using molecular biological methods, the team has succeeded in fusing the hydrogenase of the cyanobacterium Synechocystis sp. PCC 6803 directly with the photosystem I (PS I) enzyme complex, which is crucial for photosynthesis. PS I supplies energy-rich electrons that the hydrogenase needs for its work.
Under normal circumstances, the bacterial cell quickly consumes the resulting hydrogen itself. As the researchers reported in the journal Nature Energy, the mutants successfully produce photosynthetic hydrogen and, as hoped, do not subsequently absorb it again.
The simultaneously produced oxygen inhibits the activity of the hydrogenase. Therefore, the researchers currently remove the oxygen enzymatically and with the addition of glucose. However, carbohydrate metabolism and hydrogen production form a complex interplay in the cells, because hydrogenase can also use glucose for energy.
Gutekunst's team wants to decouple this part of the process so that hydrogen production is based exclusively on solar energy and the splitting of water. The vision of the Kassel researchers is to store solar energy via photosynthesis in the form of solar hydrogen. Whether the process can be developed to product maturity is not yet foreseeable at the current state of research. Gutekunst received the BMBF research award "Next Generation of Biotechnological Processes" in 2016 to promote her work.
The problem with oxygen inhibiting hydrogenase is also being addressed by the team led by Thomas Happe at the Ruhr University in Bochum. Theoretically, a single hydrogenase enzyme can produce 10,000 hydrogen molecules per second - if oxygen does not interfere with them. The researchers have succeeded in producing the part of the hydrogenase that is crucial for this reaction, the so-called cofactor, and providing it with a protective shell of proteins. In the long term, this shell is to be minimized so that the cofactor is bound to a simple, stable structure that is insensitive to oxygen. Together with teams from the Technical University of Munich, the Max Planck Institute for Chemical Energy Conversion and the CNRS Marseille, a milestone has now been reached and a hydrogenase has been presented that, embedded in a polymer, can split water into hydrogen using electricity - and can do so for several weeks in the presence of oxygen.
Page 4 of 7
Biomass fermentation: Fermenting residues to H2
Biomass is a renewable source of hydrocarbons, on the basis of which hydrogen can be produced. The term biomass fermentation refers to the microbial metabolization of biomass, which includes, for example, fermentation. The following processes are relevant for hydrogen production:
Photofermentation: This metabolic pathway is used by some photosynthetic bacteria, for example purple bacteria, when nitrogen is scarce. Sunlight serves as the energy source for hydrogen production, and the electron source is organic acids or hydrogen sulfide, for example, which are formed from the decomposition of organic substances. Electrons from the enzyme complexes photosystem I and photosystem II react with the protons to form hydrogen. This reaction is catalyzed by the enzyme nitrogenase. Compared to the dark fermentation described later in this dossier, photofermentation is significantly more effective and efficient. The process is also less sensitive to oxygen.
Dark fermentation: This alternative metabolic route of hydrogen production does not require light and takes place under anaerobic conditions, i.e., in the absence of oxygen. Organic molecules, such as sugars or organic acids, serve instead as both the energy source and the electron source. Anaerobic bacteria such as the clostridia, as well as some archaea, engage in dark fermentation. In this process, complex carbon compounds are degraded to simpler compounds by hydrolysis in the microorganisms, producing hydrogen and CO2. Although the process has a high conversion rate, it results in a rather low hydrogen concentration, which requires purification of the gas. On the other hand, the reaction is not limited by oxygen. A study by the University of Erlangen-Nuremberg classifies this process at a TRL level of 6, i.e., the technology is being tested in an industrially relevant environment.
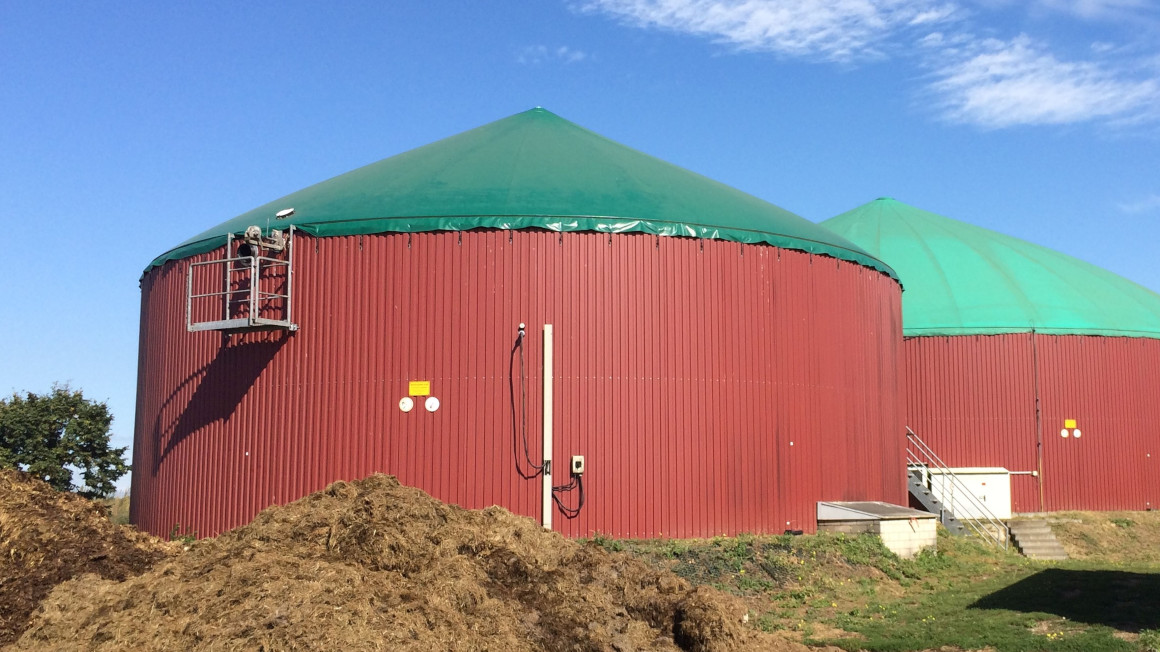
Publicly funded research activities
In the HyPerFerment (Hydrogen Per Fermentation) project, funded by the EU, Investitionsbank Sachsen-Anhalt and the German Federal Ministry of Economics, Fraunhofer researchers are investigating microbial dark fermentation in biogas plants. They are using residual materials as substrate. Under oxygen-free conditions, certain bacteria ferment organic substances in roughly equal parts to hydrogen and CO2. The advantage of this process is that neither methane nor interfering components such as hydrogen sulfide are produced. However, the decomposition of organic substances by dark fermentation is incomplete. In the research project, it is therefore to be coupled with conventional biogas synthesis. In this process, the organic residues are fermented to methane and CO2. The latter can be separated or chemically recycled. Methane is used as an energy source for heating. Because many synergies are created when the two processes are coupled, the overall balance is particularly advantageous.
The German Federal Ministry of Economics and Technology is funding the research project "HyTech - Biological Hydrogen Production for a Sustainable Energy Economy" by researchers at Münster University of Applied Sciences and the Berlin-based start-up BlueMethano GmbH. Here, the possibilities for optimizing the dark fermentation process are to be investigated. As in a biogas plant, hydrogen is extracted from biomass by means of microorganisms. In the absence of light and oxygen, bacteria convert organic substances into hydrogen and acids. This is similar to the biogas process, but it still includes methane formation. In dark fermentation, this stage takes place in a second reactor.
Page 5 of 7
Electrobiochemistry: electricity-based biohydrogen
The young field of electrobiotechnology aims to link microbial and electrochemical material conversions. This would allow electricity from renewable energy sources to be used to synthesize chemicals and energy carriers. As part of such a power-to-X process, hydrogen can also be produced biobased - with the help of a so-called microbial electrolysis cell.
The most important biological actors in such a process are electrochemically active bacteria. They decompose organic compounds, for example from wastewater, initially generating CO2 and electrons. The electrons flow from the anode to the cathode, where they react with hydrogen atoms and thus release protons. However, this method of hydrogen production requires a continuous supply of energy to maintain the voltage. The big advantage is that residual biomass streams from wastewater treatment can be used in this process.
A very recent development is the microbial photoelectrochemical cell. Microorganisms colonize the anode and generate electrons here electrochemically from organic compounds. A photoelectrochemical process driven by sunlight ultimately results in water molecules being separated into hydrogen and oxygen. Because this process also has enormous sustainability potential, it is increasingly becoming the focus of research. However, there are only a few research groups actively pursuing this path. This is because growing biofilms inhibit the process.
Publicly funded research activities
The project "BioDME - Sustainable synthesis of the energy carrier dimethyl ether from wastewater", funded by the German Federal Ministry of Education and Research (BMBF), is part of electrobiochemistry research. Wastewater treatment requires a lot of energy to remove organic residues. In contrast, the researchers want to harness the energy contained in wastewater and use it for the synthesis of valuable substances. In microbial fuel cells, certain bacteria are to break down the organic substances and generate electricity at the same time. If an additional voltage is applied, hydrogen can be produced directly, with far less energy required than in classical electrolysis, since the bacteria extract part of the energy from the wastewater. Theoretically, the hydrogen could now be stored or transported away. In the project, however, it is chemically processed directly into dimethyl ether.
The production of green hydrogen from residual materials was also the goal of the BMBF-funded joint project "Development of Cascade Reactors for the Conversion of Biogenic Waste Streams into Hydrogen and Propionate" (RECICL). The researchers developed two biotechnological processes to convert biogenic residual and waste material streams into platform chemicals. First, the process produces acetate, butyrate and propionate from the waste. In a microbial electrolysis cell, acetate and butyrate then react to form CO2 at the anode, while molecular hydrogen is produced at the cathode. The remaining propionate is concentrated by filtration for further use. In an extension of this process chain, hydrogen, CO2 and propionate are used as nutrients for the microbial production of butanediol.
Page 6 of 7
Research landscape on biobased hydrogen
Research on bio-based hydrogen is still a niche in Germany, mostly limited to basic research, and many research institutions currently focus their efforts on the more easily available green hydrogen from renewable energies. Nevertheless, some research teams are doing groundbreaking work here in particular:
- At Ruhr-Universität Bochum, researchers led by Thomas Happe, Wolfgang Schuhmann and Marc Nowaczyk are working on the optimization of hydrogenases and the use of algae as production organisms.
- At the University of Bremen, the focus is on microbial electrolysis.
- At Kiel Christian Albrechts University, Miriam Perner's team is pioneering research into cyanobacteria for the production of hydrogen by microbial photosynthesis.
- At TU Berlin, Oliver Lenz's team is working with hydrogenases from oxyhydrogen bacteria. Lenz's former colleague Lars Lauterbach will continue his work on biobased hydrogen as a professor at RWTH Aachen University.
- Researchers at the Zittau/Görlitz University of Applied Sciences are also working on biobased hydrogen.
- Bremerhaven has its own hydrogen competence center, which also conducts research into biobased hydrogen.
- The Leibniz Institute for Catalysis (LIKAT) is investigating how hydrogen can be produced biotechnologically from biogenic residues.
- Similar to Bremerhaven, Leuna has also set up a "Hydrogen Lab" in which numerous aspects relating to hydrogen are being researched.
- At Münster University of Applied Sciences, Elmar Brügging and his team are researching dark fermentation.
- At the Helmholtz Centre for Environmental Research (UFZ) in Leipzig, Andreas Schmid coordinates research on stable reaction systems for hydrogen production by cyanobacteria.
- At the University of Kassel, Kristin Gutekunst's team is researching hydrogen production using biotechnologically processed cyanobacteria.
- At the Max Planck Institutes, teams led by Wolfgang Lubitz at MPI Mülheim, Nicole Dubilier at MPI Bremen and Saigo Shima at MPI Marburg are researching biobased hydrogen.
- Last but not least, a cross-institute hydrogen network has been formed within the Fraunhofer-Gesellschaft, in which biobased hydrogen is one of many topics. The Fraunhofer IFF, for example, is involved.
International cooperations
German research institutions are also cooperating internationally in partnerships funded by the German Federal Ministry of Education and Research. For example, there is a program for funding individual or joint projects on the production of green hydrogen by means of biomass gasification or fermentation with participants from New Zealand. Research projects carried out jointly with research institutions from Central Asia are also funded around hydrogen production from or by means of biomass, for example the development of a catalytic hydrogen generator from bio raw materials or biotechnologies to produce hydrogen from food waste.
Page 7 of 7
Further information
Contents only available in German
These studies and statements served as the knowledge base for the topic dossier on biohydrogen