Nanotechnology allows for larger 3D structures
A new technique enables Hendrik Dietz at TU Munich to build DNA origami structures the size of viruses, while also reducing the cost of production substantially.
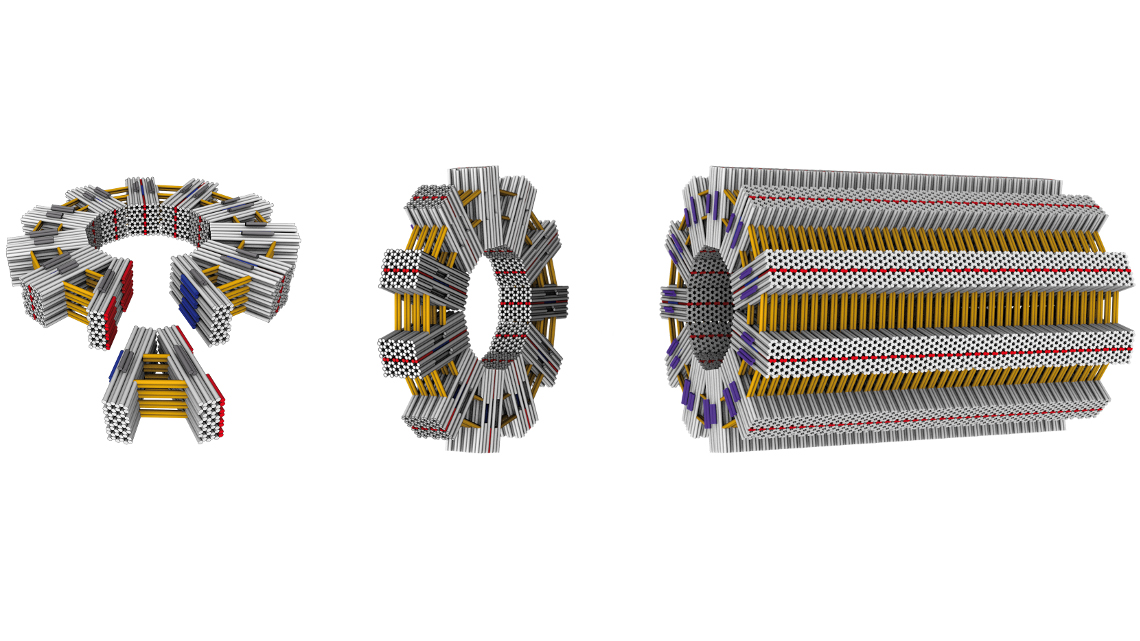
Using the "DNA-origami-technique" researchers can fold single DNA strands into a three-dimensional double-stranded structure. Biophysicist Hendrik Dietz, Professor of Biomolecular Nanotechnology at TU Munich, is an expert of this field and has now developed a new way to make the tiny DNA origami structures larger by transfering viral construction principles to DNA origami technology. This enables him and his team to design and build much larger structures than before – now on the scale of viruses and cell organelles. The researchers published their findings in the journal Nature.
V-shaped nano-objects autonomously attach to each other
To build these larger structures the researchers first created V-shaped nano-objects. These have shape-complementary binding sites on their sides, allowing them to autonomously attach to each other while floating in a solution. Depending on the opening angle, they form “gears” with a controlled number of spokes. “We were thrilled to observe that, almost without exception, rings formed as defined by the opening angle,” says Dietz. “Decisive for the ability to build objects of this size and complexity is the precision and rigidity of the individual building blocks. We had to reinforce individual elements with crossbars, for instance.”
Building nanotubes one micrometer long
To further exploit the construction principle, the team created new building blocks that had “glue joints” not only on the sides, but also slightly weaker ones on the top and bottom. This allows the “nano-gears” to form long tubes using the additional docking sites in a second step. “At lengths of one micrometer and a diameter of several hundred nanometers, these tubes have reached the size of some bacteria,” explains Hendrik Dietz. “And we can use the architecture of individual elements to determine features of the overall structure.”
For future applications the researchers hope to use polyhedral “cage-like” structures that could release target-specific treatment.
Reducing manufacturing costs
However, to date, manufacturing processes have limited the scope of application to those requiring only small amounts of material. The fact that only a few micrograms can be manufactured with conventional methods precludes many potential medical and materials science applications.
The bottleneck is the short staple strands that must be chemically produced base by base. The main strand, which is obtained from bacteriophages, on the other hand, can be produced on a large scale using biotechnological processes.
That is why Dietz and his team refined the so-called DNA enzymes. These are DNA strands that break apart at specific positions when exposed to a high concentration of zinc ions.
Both the main strand and the secondary strand, comprising DNA enzymes and the staple sequences, were successfully produced using a high cell density process with bacteria. The process is scalable and thus amenable to high volume production of the main strands and staples. Increasing the zinc ion concentration after DNA isolation releases the short staple sequences, which then fold the main strand into the desired shape.
jmr